Recording neurons and neuronal networks in action is crucial for understanding brain functions. Fluorescent voltage indicators follow changes of plasma membrane potential that accompany neuronal activity. In this study, Ludwig et al. advanced the development of a competent voltage indicator by characterizing exceptionally bright and stable single-particle nanosensors that respond to fluctuations of neuronal membrane potential.
These nanosensors are based on tiny (4x10 nm) semiconductor nanorods spontaneously inserted into the plasma membrane of neurons. The overall brightness and the emission wavelength of nanorods depend directly on changes in the external electric field consistent with the quantum-confined Stark effect. The authors monitored photoluminescence of nanorods using a wide-field microscope equipped with an emission splitter and a fast and sensitive CMOS camera. To facilitate the insertion of nanorods into the plasma membrane, they functionalized their surface with brain lipids (extracted from porcine).
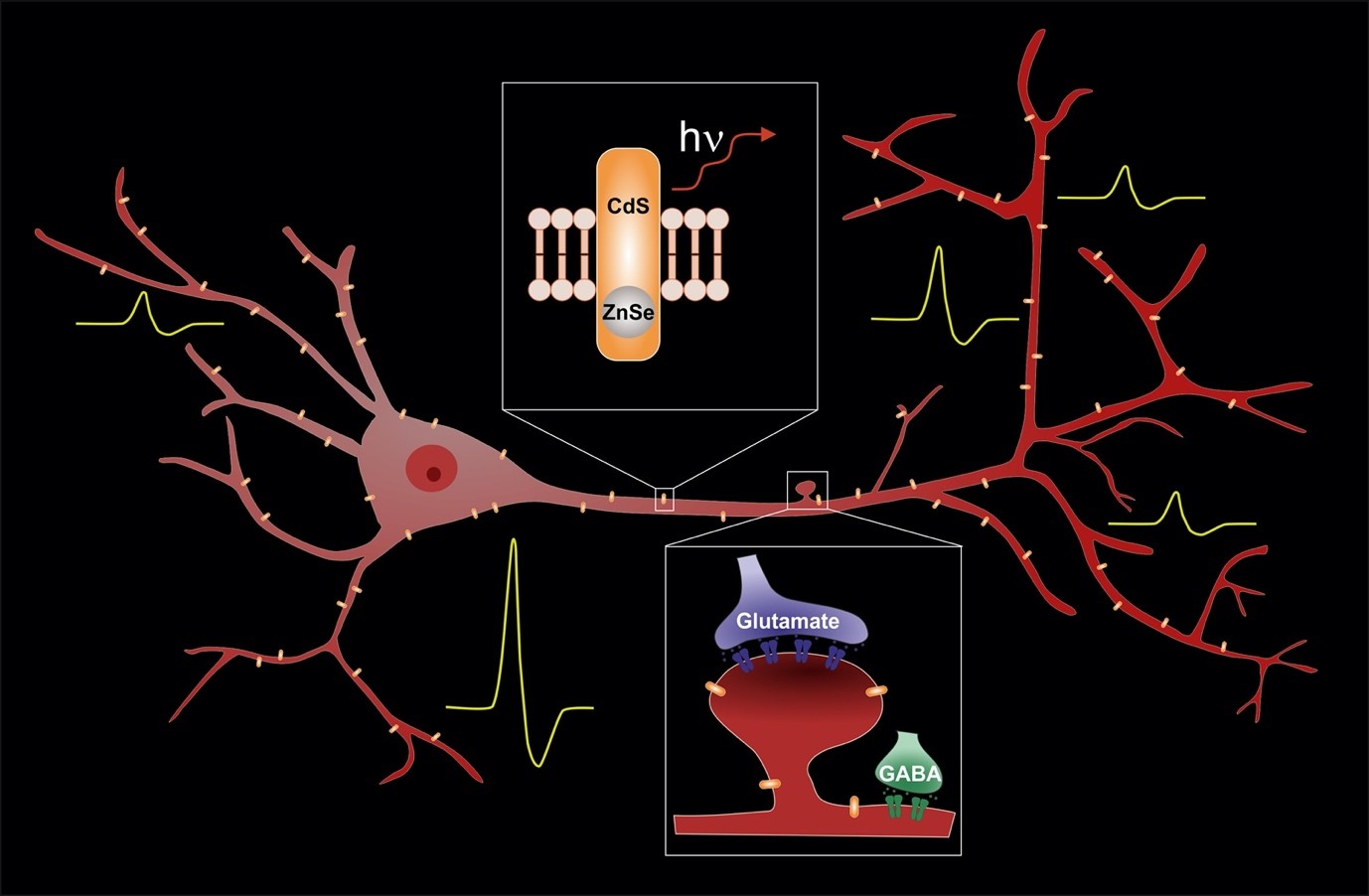
The development of voltage nanosensors is a result of an international and interdisciplinary collaboration between chemists, physicists, and biologists from the USA, Israel, Germany, and France, enabled by HFSP (grant #RGP0061) and ERC (#NVS). We explored a theoretical basis for using asymmetric semiconductor nanorods for membrane potential monitoring (Park and Weiss, 2017), characterized the nanorods’ response to external voltage modulation using arrays of electrodes (Kuo et al., 2018, Bar-Elli et al., 2018), and demonstrated the validity of the nanorod approach for recording membrane potentials in living cells, including neurons (Park et al., 2018, Park et al., 2019, Ludwig et al., 2020).
We also established a workflow to process photoluminescent signals from nanorods embedded into neuronal membranes confirming that nanorods possess sensitivity enough to detect changes in the membrane potential at a single-particle level. In contrast to in-between-electrodes preparations, we found that in living cells nanorods exhibited fluctuations of voltage sensitivity likely attributed to their unstable insertion into the membrane. To overcome the problem, further optimization of the nanorod surface is needed to stabilize the particles inside the plasma membrane.
Our study shows the potential of voltage nanosensors for the detection of neuronal activity with unprecedentedly high spatial and temporal resolution. Such sensors open a possibility for super-resolution voltage imaging from confined subcellular locations (such as individual synapses, spines, dendrites) and hence providing neuroscientists with a versatile novel and powerful tool to study the brain in action.